Some recent highlights
Biomolecular simulation: introduction, status, progress and prospects, A model solution to depression, and Rewriting biochemistry textbooks, and Chemical accuracy in QM/MM calculations on enzyme-catalysed reactions.
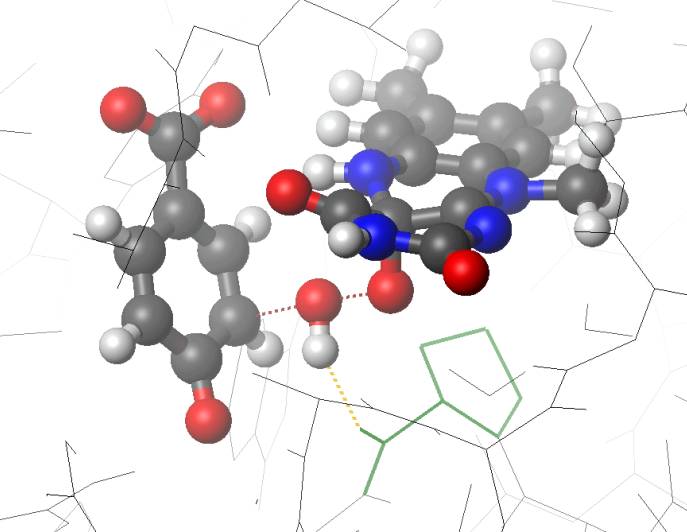
How a biological reaction happens. This picture shows the transition state in the enzyme para-hydroxybenzoate hydroxylase, as found by QM/MM modelling. An OH group (centre) is transferred from a flavin cofactor (right) to the substrate (left). The transition state is stabilized by a hydrogen bond interaction with a key group in the enzyme (shown as a dotted line). This catalytic effect helps to make the biodegradation of aromatic compounds efficient. Para-hydroxybenzoate hydroxylase (PHBH) is an important enzyme in the microbial biodegradation of a wide variety of aromatic chemicals, including pollutants and lignin, a major component of wood and so among the most abundant of all biopolymers. PHBH acts by oxygenating its substrates (adding a hydroxyl (OH) group to the aromatic ring), and is a key member of an important class of oxygenase enzymes.
Quantum tunnelling in enzyme-catalysed reactions
Modelling of aromatic amine dehdrogenase demonstrates that in this enzyme reaction tunnelling is very important and that, crucially, the tunnelling is promoted only by short-range molecular motion at the active site. Long-range flexing of the rest of the protein molecule plays no direct role in the process. The findings have sparked a keen debate among biologists about the fundamental nature of enzymes, and the role of conformational changes in the bulk protein molecule.
You can read more about this work in The Economist (a copy of the article is available here), Science, Seed Magazine, and Chemistry World.
Most biochemical
reactions
depend on enzyme catalysis, and understanding how enzymes 'work' at the
molecular level is a fundamental problem. Enzymes are remarkably
efficient and specific catalysts, but despite intensive experimental investigations,
the detailed origins of their rate accelerations remain unclear.
This question is of crucial importance in biology, and also for the development
of protein catalysts for practical applications. Better understanding
is vital for analysing the activities of mutant or
designed proteins, and for the design of inhibitors
as pharmaceutical lead compounds.
Citrate
synthase
Computer
simulations are a good way to study enzyme reactions. They
can provide information which is often inaccessible experimentally, such
as details of unstable species (for example transition states and reaction
intermediates) and on energetic contributions to catalysis. Simulating
an enzyme reaction is a challenging problem, and requires the use of specialized
techniques. An effective approach is to use combined
quantum mechanical/molecular mechanical (QM/MM)
methods. Molecular mechanics methods can be used to study
protein conformational changes, dynamics and binding, but generally they
can't be applied to processes involving the breaking or making of chemical
bonds. For chemical reactions a quantum mechanical description is
needed, which can be achieved by the combined QM/MM approach. The
small QM region contains the groups involved in the reaction (e.g. the
catalytic residues and the substrate(s)) and is influenced by the surroundings
(represented more simply by standard molecular mechanics), so including
the effects of the environment. In this way the reaction in an enzyme
can be studied, and contributions of individual groups can be analysed.
The central theme of my work
is the use of simulation methods to investigate enzyme mechanism and dynamics
(in several cases in active collaboration with experimental studies).
Programming expertise is not required for most of the present work. Projects
offered include:
-
Mechanisms of glycosidases. Specific
hydrolysis of glycosidic bonds of carbohydrates is required for a wide
variety of metabolic processes, and is catalysed by an important family
of enzymes, the glycosidases. Simulations are being used to investigate
substrate binding, and unresolved mechanistic questions, using structures
of enzyme-inhibitor complexes.
-
Aspartate beta semi-aldehyde dehydrogenase (ASADH) is a bacterial biosynthetic
enzyme, and a target for the development of anti-bacterial
agents. We are examining the mechanism of ASADH and inhibitor
binding in collaboration with Dr.
Andrea Hadfield of the Dept.
of Biochemistry, who is studying the enzyme by X-ray crystallography.
-
We are working to understand differences in activity and stability between
normal enzymes and those isolated from ‘thermophiles’
(organisms which thrive at high temperature). Study of the dynamics
and mechanisms of these enzymes will help elucidate the adaptations responsible
for these differences, with important implications for the design of stable,
active protein catalysts for practical synthetic and biotechnological applications
(in collaboration with experimental work at the Centre
for Extremophile Research, University of Bath).
-
Other work includes studies of biological ligand
binding, solvation and electronic effects in catalysis, and method
development for the study of enzyme reaction dynamics.
Some references (please contact me if you would
like reprints):
-
L. Masgrau, A. Roujeinikova, L.O. Johannissen, P. Hothi, J. Basran, K.E. Ranaghan, A.J. Mulholland, M.J. Sutcliffe, N.S. Scrutton & David Leys Atomic Description of an Enzyme Reaction Dominated by Proton Tunneling Science 14 April 2006: Vol. 312. no. 5771, pp. 237 - 241. DOI: 10.1126/science.
-
J.C. Hermann, L. Ridder, H.-D. Höe & A.J. Mulholland 'Molecular mechanisms of antibiotic resistance: QM/MM modelling of deacylation in a class A beta-lactamase, Org. Biomol. Chem., 2, 206-210 (2006).
-
N. Strickland, A.J. Mulholland & J.N. Harvey 'The Fe-CO Bond Energy in Myoglobin: A QM/MM Study of the Effect of Tertiary Structure' Biophys. J., 90, L27-29L (2006).
-
A. Lodola, M. Mor, J.C. Hermann, G. Tarzia, D. Piomelli and A.J. Mulholland 'QM/MM modelling of oleamide hydrolysis in fatty acid amide hydrolase (FAAH) reveals a new mechanism of nucleophile activation' Chem. Commun. 4399-4401 (2005).
-
C.M. Bathelt, A.J. Mulholland and J.N. Harvey 'QM/MM Studies of the Electronic Structure of the Compound I Intermediate in Cytochrome c Peroxidase and Ascorbate Peroxidase' Dalton Trans., 21,3470-3476 (2005).
-
A.J. Mulholland 'Modelling enzyme reaction mechanisms, specificity and catalysis' Drug Discovery Today 10, 1393-1402 (2005).
-
F. Claeyssens, K.E. Ranaghan, F.R. Manby, J.N. Harvey & A.J. Mulholland, 'Multiple high-level QM/MM reaction paths demonstrate transition-state stabilization in chorismate mutase: correlation of barrier height with transition-state stabilization'. Chem. Commun. 5068-5070 (2005).
-
C.M. Bathelt, J. Zurek, A.J. Mulholland & J.N. Harvey 'Electronic Structure of Compound I in Human Isoforms of Cytochrome P450 from QM/MM Modeling' J. Am. Chem. Soc., 127, 12900-12908 (2005).
-
J.C. Hermann, C. Hensen, L. Ridder, A.J. Mulholland & H.-D. Höe 'Mechanisms of antibiotic resistance: QM/MM modeling of the acylation reaction of a class A beta-lactamase with benzylpenicillin'. J. Am. Chem. Soc., 127, 4454-4465 (2005).
-
T. Rungrotmongkol, S. Hannongbua & A.J. Mulholland 'Mechanistic Study of HIV-1 Reverse Transcriptase at the Active Site Based on QM/MM Method' J. Theoretical and Computational. Chemistry 3, 491-500 (2004).
-
B. Szefczyk, A.J. Mulholland, K.E. Ranaghan & W.A. Sokalski 'Differential Transition State Stabilization in Enzyme Catalysis: Quantum Chemical Analysis of Interactions in the Chorismate Mutase Reaction and Prediction of the Optimal Catalytic Field.' J. Am. Chem. Soc. 126, 16148-16159 (2004).
-
C.M. Bathelt, L. Ridder, A.J. Mulholland & J.N. Harvey 'Mechanism and Structure-Reactivity Relationships for Aromatic Hydroxylation by Cytochrome P450' Organic and Biomolecular Chemistry, 2, 2998-3005 (2004).
-
K.E. Ranaghan & A.J. Mulholland 'Conformational effects in enzyme catalysis: QM/MM free energy calculation of the 'NAC' contribution in chorismate mutase' Chem. Commun. (10), 1238-1239 (2004).
-
K.E. Ranaghan, L. Ridder, B. Szefczyk, W.A. Sokalski, J.C. Hermann, and A.J. Mulholland 'Transition state stabilization and substrate strain in enzyme catalysis: ab initio QM/MM modelling of the chorismate mutase reaction' Organic and Biomolecular Chemistry 2, 968-980, (2004).
-
C.M. Bathelt, L. Ridder, A.J. Mulholland & J.N. Harvey 'Aromatic hydroxylation by cytochrome P450: Model calculations of mechanism and substituent effects' J. Am. Chem. Soc., 125, 15004 -15005 (2003).
-
K.E. Ranaghan, L. Ridder, B. Szefczyk, W.A. Sokalski, J.C. Hermann, and A.J. Mulholland 'Transition state stabilization and substrate strain in enzyme catalysis: ab initio QM/MM modelling of the chorismate mutase reaction' Organic and Biomolecular Chemistry (2004) 2, 968-980
-
J. Zurek, A.L. Bowman, W.A. Sokalski & A.J.Mulholland 'MM and QM/MM Modeling of Threonyl-tRNA Synthetase: Model Preparation, Testing and Simulations', Structural Chemistry, 15, 405-414 (2004).
-
K.E. Ranaghan & A.J. Mulholland 'Conformational effects in enzyme catalysis: QM/MM free energy calculation of the 'NAC' contribution in chorismate mutase' Chem. Commun. (2004) (10), 1238-1239
-
C.M. Bathelt, L. Ridder, A.J. Mulholland & J.N. Harvey 'Mechanism and Structure-Reactivity Relationships for Aromatic Hydroxylation by Cytochrome P450' Organic and Biomolecular Chemistry, (2004) 2, 2998-3005.
-
J.C. Hermann, L. Ridder, A.J. Mulholland, and H.-D. Hoeltje, Identification of Glu166 as the General Base in the Acylation Reaction of Class A beta-Lactamses through QM/MM Modeling J. Am. Chem. Soc.
125, 9590-9591 (2003)
-
L. Ridder and A.J. Mulholland, Modeling biotransformation reactions by combined quantum mechanical/molecular mechanical approaches: from structure to activity Curr. Topics in Medicinal Chemistry.
3, 1241-1256 (2003)
-
K. Ranaghan, L. Ridder, B. Szefczyk, W. A. Sokalski and A.J. Mulholland, Insights into enzyme catalysis from QM/MM modelling: transition state stabilization in chorismate mutase Mol. Phys.
101, 2695-2714 (2003)
-
L. Ridder, J.N. Harvey, I.M.C.M. Rietjens, J. Vervoort and A.J. Mulholland, Ab initio QM/MM Modeling of the Hydroxylation Step in p-Hydroxybenzoate Hydroxylase J. Phys. Chem. B
107, 2118-2126 (2003)
-
L. Ridder, I.M.C.M. Rietjens, J. Vervoort and A.J. Mulholland, Quantum Mechanical/Molecular Mechanical Free Energy Simulations of the Glutathione S-Transferase (M1-1) Reaction with Phenanthrene 9,10-Oxide J. Am. Chem. Soc. 124, 9926-9936 (2002)
-
A.J. Mulholland, P.D. Lyne and M. Karplus, Ab Initio QM/MM Study of the
Citrate Synthase Mechanism. A Low-Barrier Hydrogen Bond is not
Involved J. Am. Chem. Soc.
122, 534-535 (2000)
-
A.T. Hadfield and A.J. Mulholland, Active Site Dynamics of ASADH-
A Bacterial Biosynthetic Enzyme Int. J. Quant. Chem.,
Biophys. Q. 73, 137-146 (1999)
-
L. Ridder, A.J. Mulholland, J. Vervoort and I.M.C.M. Rietjens, Correlation
of Calculated Activation Energies with Experimental Rate Constants for an
Enzyme-Catalyzed Aromatic Hydroxylation J. Am. Chem. Soc.
120, 7641-7642 (1998)
-
A.J. Mulholland and W.G. Richards, Modeling Enzyme Reaction Intermediates
and Transition States: Citrate Synthase J.Phys.Chem. B
102, 6635-6646 (1998)
-
A.J. Mulholland, G.H. Grant and W.G. Richards, Review: Computer modelling
of enzyme catalysed reaction mechanisms. Protein Engineering
6, 133-147 (1993)
-
P.D. Lyne, A.J. Mulholland and W.G. Richards, Insights into Chorismate
Mutase Catalysis from a Combined QM/MM Simulation of the Enzyme Reaction.
J. Am. Chem. Soc. 117, 11345-11350 (1995)
-
A.J. Mulholland and M. Karplus, Simulations of enzymic reactions,
Biochem. Soc. Trans. 24, 247-254 (1996)
-
A.J. Mulholland and W.G. Richards, Acetyl-CoA Enolization in Citrate
Synthase: A Quantum Mechanical/Molecular Mechanical (QM/MM) Study,
Proteins: Structure, Function, and Genetics 27, 9-25 (1997)
-
L.Ridder, A.J. Mulholland, I.M.C.M. Rietjens and J. Vervoort, A Quantum Mechanical/Molecular Mechanical Study of the Hydroxylation of Phenol and Halogenated Derivatives by Phenol Hydroxylase,
J. Am. Chem. Soc. 122, 8728-8738 (2000)
Back to School
of Chemistry Home Page